The views expressed in this paper are those of the writer(s) and are not necessarily those of the ARJ Editor or Answers in Genesis.
Abstract
The palo verde tree, Parkinsonia spp., is well known to residents of the desert southwest and Mexico, yet there are few studies of it in the technical literature. As a drought-resistant, woody plant, it is unique in several ways, most notably its bright green trunk, stems, and petioles where most photosynthesis is performed. Stem photosynthesis is, however, not unique to this desert plant. Other unusual features of the palo verde plant include extensive root systems, heavily cutinized epidermis and vascular bundles, sunken stoma, very small leaves (leaflets), drought-deciduous behavior, idioblasts, C4 photosynthesis, and the plentiful accumulation of storage products, oil, starch, and calcium oxalate. These features make this plant uniquely fitted to thrive in arid, desert biomes. More work is required to clearly isolate and describe such tissues as the cambium, primary and secondary phloem and xylem bundles, and the extent of internal cutinization of this unusual woody plant. We conclude that the sum of these unique features make it purposefully fitted to the biomes in which it grows.
Keywords: Palo verde, Parkinsonia, Cercidium, drought tolerance, drought deciduous, desert plants, ultrastructure, electron microscope, plant design
Introduction
The palo verde tree (well known to residents of Texas, Arizona, New Mexico, Mexico, as well as Central and South America) has bright green trunks, stems, and leaves and is perfectly fitted for life in the harsh Southwest. The bright green nature of the trunk, stems, and petioles of Parkinsonia is undoubtedly the reason why this tree is known as the palo verde (“green stick” in Spanish). It is extremely drought tolerant, thrives on little water, and is even considered a weed in many communities. It is drought deciduous, meaning that it drops its leaflets during certain, particularly dry periods, and it also employs stem photosynthesis, which means that most photosynthesis takes place in the petioles, stems, and trunk, and not in the leaves.
Palo verde has variants found on Hawaii, the Galapagos Islands, and southern Africa, as well as in India. In Australia, where it was introduced as an ornamental and shade tree in the year 1900, it has become a widely spread invasive, requiring the introduction of several insects to serve as biological controls to limit further spread. Palo verde has also been reported from the Caribbean as well as Central and South America. In Florida, it is prized as an ornamental. In 1966, it was named the city tree of Miami.
The palo verde was also made the official state tree of the state of Arizona in 1954, although two distinct species were lumped together by the state as its tree: Parkinsonia microphylla and P. florida. Both trees have green trunks and stems but the blue palo verde has more of a blue-green tint. The Arizona state website continues to refer to P. florida as Cercidium foridum, an earlier genus and species name which persists in the literature.
It may be that the widespread usage of Parkinsonia as a landscape ornamental in Arizona (Dimmit 1987, 2014; Schuch and Kelly 2008, 2012), New Mexico, Florida and outside of the United States has contributed to its familiarity. However, little besides the technical aspects of landscape management is known about the unique characteristics of this remarkable plant. These characteristics reported here are, in our minds, indicators of planning and purpose. This unique collection of characteristics allows it to thrive in environments which might kill many other well-known ornamental plants. The only alternative to a purposeful explanation of its origin is, of course, descent with modification, which we find lacking as a reasonable explanation for the collection of characteristics found in palo verde.
Materials and Methods
Stems and petioles of Parkinsonia florida (blue palo verde tree) were collected from sites in Arizona, fixed in 2% glutaraldehyde, postfixed in osmium, dehydrated through a graded series of acetone and embedded in Embed 812 (Electron Microscopy Sciences, Hatfield, Pennsylvania). Leaves were not studied. Tissue fixation, postfixation, and dehydration were performed in a laboratory microwave (Pelco Biowave, Ted Pella, Inc., Redding, California).
Embedded stems were selected for light microscopy (thick) sectioning and transmission electron microscopy (TEM) thin sectioning. Thick sections were collected on glass slides and stained with Epoxy Tissue Stain (EMS), which imparts blue and blue-related colors to the tissues and to the embedding polymer. Gold sections were collected with copper grids, stained in lead citrate and uranyl acetate, and imaged in an AEI 801 TEM. Other sections were affixed to aluminum stubs, sputter coated with gold and examined on a Hitachi S 2500 scanning electron microscope.
Here we explore some of the unique anatomical features of the palo verde stem. We intend to discuss morphology and fitness of leaves and petioles in a future report.
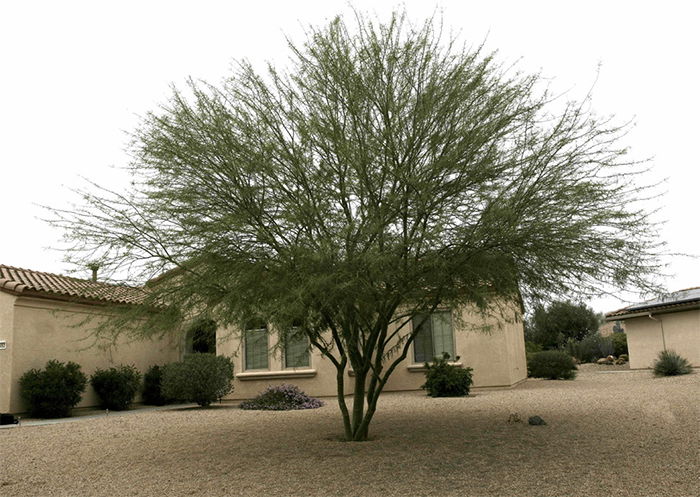
Fig. 1. Palo verde used as an ornamental tree.
Results
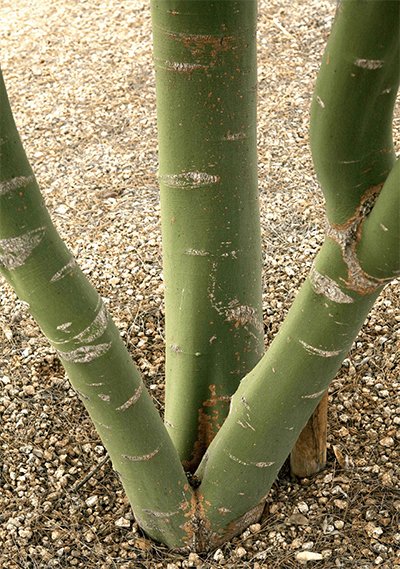
Fig. 2. Trifurcating trunk of palo verde. Note green color from ground up, indicating presence of chlorophyll in this woody stem. Horizontal light colored ovals are scars from the locations where leaves previously grew.
There is a paucity of anatomical reports on Parkinsonia and Cercidium, thus a need for further work on the anatomy of this interesting plant exists. We could find only one study which elucidates the ultrastructure of leaves, stems, roots, and seeds of the palo verde (Scott 1935), but it lacks any study of petioles, which perform a good deal of the photosynthesis. Furthermore it lacks the necessary micrographs for a complete understanding of anatomy. It focused on only two of the 15 or so varieties of the palo verde.
Palo verde is commonly used as an ornamental tree in housing and city land improvement in the southwest (figs. 1 and 2), thus it represents a plentiful source of specimen material. The leaves of P. florida are doubly compound and many leaflets and sub leaflets are attached to each petiole (figs. 3 and 4). All are the same asparagus green color. Hair cells (fig. 3) are abundant on stems and leaves but rarely survived processing for microscopy. Stem ultrastructure agrees somewhat with results published by Scott (1935), although she studied analogs of P. florida. crystals were found as deep as secondary xylem in contrast to Scott’s report. Interestingly the calcium oxalate crystals observed in palo verde stems took the druse form. No needle-like crystals were observed in palo verde stems.
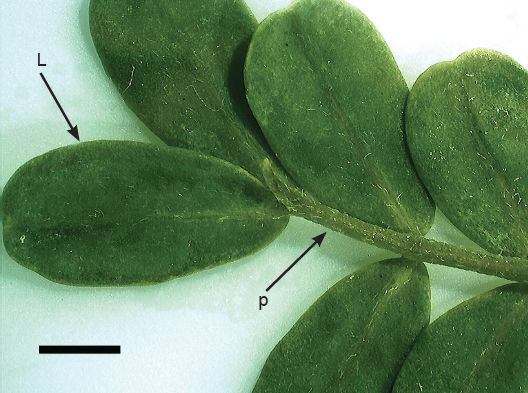
Fig. 3. Low power micrograph of palo verde leaflets (L) (microphylls), and petioles (p), which are green. The microphylls are leaflets forming doublets with palo verde’s compound leaves. Some white hairs are visible on the petioles. Scale bar = 1 mm.
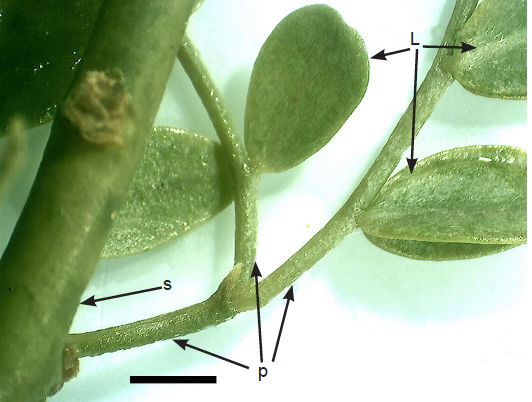
Fig. 4. Low power micrograph of leaflets (L), petioles (p), and stem (s). All contain chlorophyll, giving an identical green color. Scale bar = 1.5 mm.
The sunken guard cell pairs, idioblasts, cutined cells, and cortex are well defined (figs. 5 and 6); however, cambium, phloem, and xylem (primary and secondary) were not as well defined as hoped. Large slabs of cutin are visible in figs. 6–8. Xylem tissues were thickly lignified. Starch, stained darkly with iodine, was present in abundance, in agreement with Scott (1935) and Scott, Bystrom, and Bowler (1962), and was well distributed across phloem and xylem; however boundaries between these tissues were difficult to identify. Starch grains were highly concentrated in association with a circumferential ring of phloem (fig. 9). Many oil droplets were found deposited within photosynthetic cells and especially in chloroplasts (fig. 10). Large amounts of calcium oxalate were also deposited throughout the stem (figs. 5, 8, 10, and 11), and of note, between stoma and the outer border of the cortex (fig. 8, oblique angle). Photosynthetic cells usually contained crystals of calcium oxalate or lipids, or sometimes both, (fig. 10). Many chloroplasts were often bulging with oil droplets (fig. 10). Of note, spines also had sunken stomata (fig. 12).
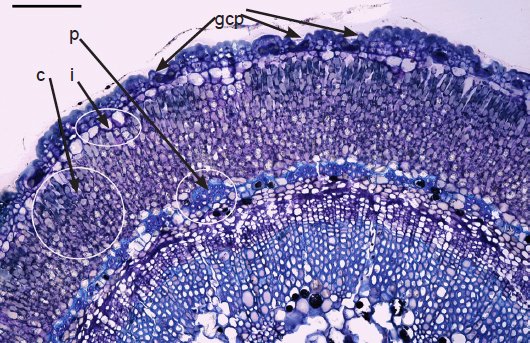
Fig. 5. 250× light micrograph of cross section of a very young palo verde stem,approximately 3 mm diameter. Note sunken guard cell pairs (gcp), thickly cutinized cells, idioblasts (i), cortex (c), and phloem (p) as part of vascular bundles. Scale bar = 0.25 mm.
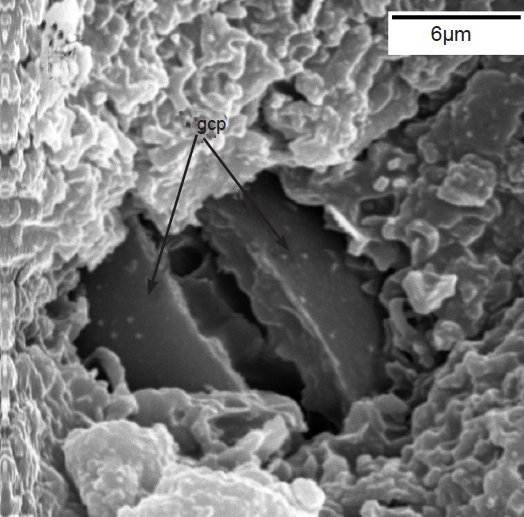
Fig. 6. Scanning electron micrograph (SEM) of sunken stoma. Note guard cell pair (gcp),(dark in color) which are sunken into thick layer of (whiter) waxy cutin on top. Scale bar = 6 microns.
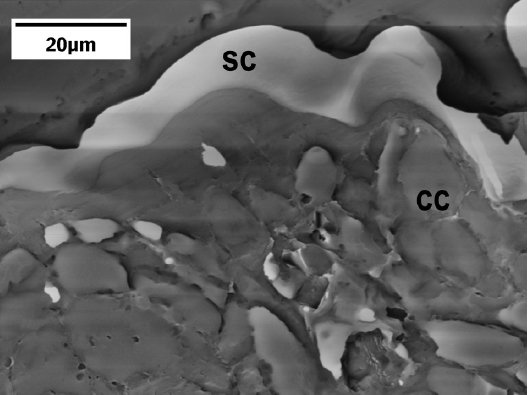
Fig. 7. SEM of palo verde epidermis showing thickened slabs of cutin (sc) and cuticular cutin (cc). Scale bar equals 20 microns.
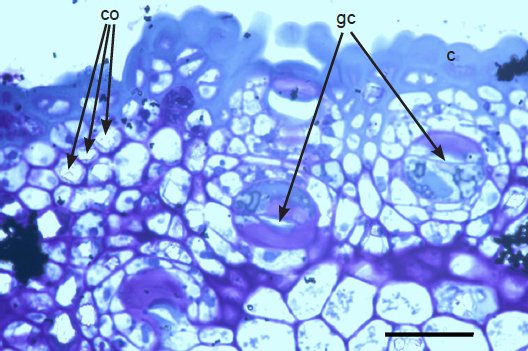
Fig. 8. 300× light micrograph of palo verde epidermis showing cutin (c), guard cells (gc), and calcium oxalate crystals (co). Scale bar = 0.1 mm.
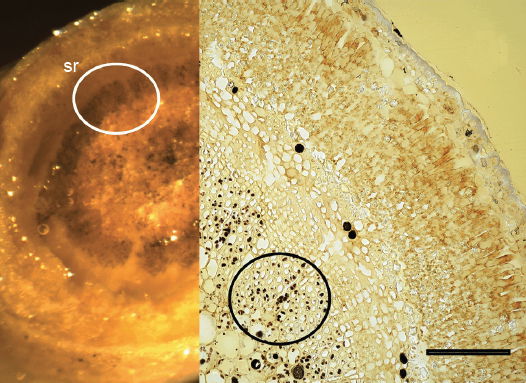
Fig. 9. 6× (left) and 300× (right) light micrographs of thick palo verde stem cross section showing stained starch ring (sr) associated with vascular bundles. Scale bar = 1 mm.
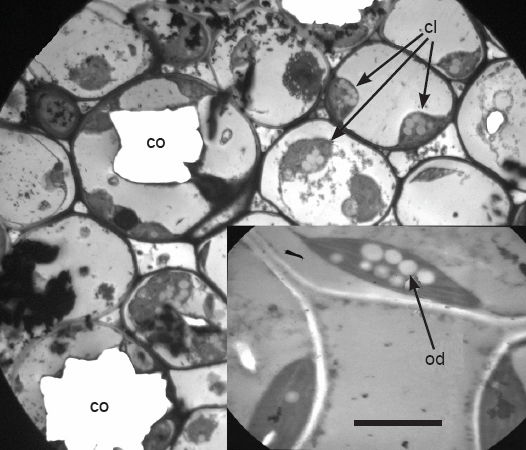
Fig. 10. Transmission electron micrograph (TEM) of photosynthetic cells with chloroplasts (cl), calcium oxalate crystals (co), and oil droplets (od). The crystals fell out of the ultra thin sections during processing, leaving the open spaces they occupied. Scale bar = 2.5 microns.
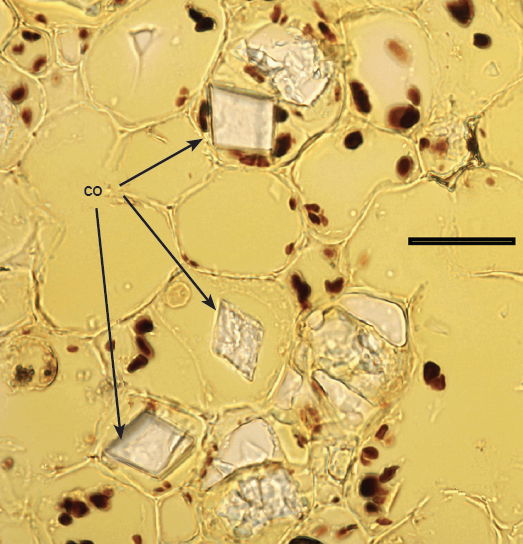
Fig. 11. 500× light micrograph of palo verde stem thin cross section showing individually stained starch grains (black) in the starch ring, and calcium oxalate crystals (co). Scale bar = 0.5 mm.
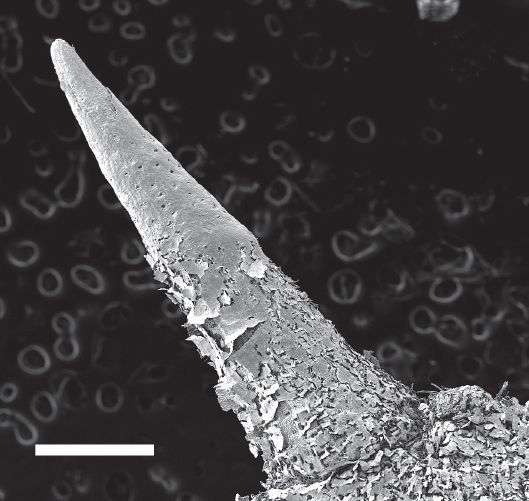
Fig. 12. SEM micrograph of Parkinsonia spine showing sunken stomata (small dark holes along top half of spine). Scale bar = 5 mm.
Discussion
Palo verde is generally found as a small tree or shrub, which lives across the dry Sonoran and Arizona deserts. The tree has a very deep root system, which allows it to locate and use deep groundwater and to survive extended periods of drought. The generous root system also allows it to survive intermittent flash floods, which are common in the southwestern deserts during summer months. Trunks may approach 10 in (25 cm) in diameter and may be decumbent or vertical. Most palo verde plants are bushes of approximately 3 ft (1 m) in height. One early report from 1856 indicates that “. . . this tree is not particularly useful. Its chief purpose is to ornament the arroyos and flood basins of the desert regions and to furnish brake blocks for desert freight-wagons.” (Rose and Standley 1912). While that observation seems to restrict palo verde to flood basins, another early report indicated that Parkinsonia preferred rocky or well-drained slopes (Cannon 1905). Perhaps early writers had not yet distinguished between Parkinsonia microphylla and P. florida which thrive in different locales.
Two major genera have been erected to encompass the different species of the palo verde tree, but there is confusion (Lersten and Curtis 1995; Polhill and Vidal 1981). There seem to be four to six species assigned to each group, or alternately, circa 13–15 species are lumped together under Parkinsonia. In their study of leaf anatomy, Lersten and Curtis (1995) offered some morphological (and therefore taxonomic) differences between Parkinsonia and Cercidium, but it is unclear if the taxonomic confusion had been resolved.
P. microphylla (the yellow or foothill palo verde) has large spines (mostly at branch terminations) which make it undesirable as a walkway or ornamental planting. It features very small leaves, which are often shed soon after the spring and winter rains subside. This variety, the slowest growing of the group, is widespread across southern Arizona and along the Colorado River in California. Small flowers exhibit five petals, mostly yellow, but some petals are white, making it a paler yellow during spring blooms (Johnson 1996; Schuch and Kelly 2008). This species of palo verde does not tolerate well-watered conditions and as a drought-deciduous tree, it drops stems as well as leaves with petioles when dry conditions persist.
P. florida, known as the blue palo verde, is often referred to as Cercidium floridum. It is faster growing than P. microphylla and is more decumbent, having trunks which often lie some distance parallel to the ground before they ascend. It has deep-yellow flowers as opposed to the paler ones exhibited by P. microphylla. Its flowers also bloom earlier than other species, and it has smaller branch thorns. P. florida appears to have a wider range than P. microphylla, but there seem to be conflicting reports of distribution in the literature. It is, however, found at non-desert elevations of 1200 m (4000 ft) and is cold tolerant to 50°F. Finally, USGS distribution charts show that P. florida (as well as P. aculeata) are native to areas mostly outside of Arizona, which is curious, as P. florida is the celebrated state tree of Arizona.
P. aculeata, also known as the Mexican palo verde, is similar to the aforementioned species. Because it is very well armed, it is no longer considered a desirable landscape tree. It develops double or triple shaped, sharp spines, and it bears large, multi-colored flowers. It is distributed across west Texas, southern Arizona, southern New Mexico, and northern Mexico. It has become invasive in Australia, forming large thickets, and has also been found in Europe, but it is sensitive to temperatures below 20°F.
There has been some success at developing hybrids, such as P. praecox, which is a three-way-cross of P. aculeata, P. microphylla, and P. florida. It is a hardy plant with no thorns and can tolerate cold temperatures down to 15°F.
For the purposes of this paper, the various members of this hybrid group will be referred to as palo verde.
Palo verde was used by native Americans as food (parched seeds) and in some instances, the extracts of bark, leaves, flowers, and fruits have been used in herbal medicines to treat arthritis and to stimulate nerves (Liogier 1990).
Little work has been done on palo verde tree anatomy/ultrastructure or otherwise describing stem features and other characteristics. Few papers of it exist in the literature. The notable exception that it is often featured in horticultural and ornamental landscape magazines, thus it has received some interest for xeric use in Arizona, New Mexico, and west Texas neighborhoods.
Drought-Deciduous Behavior
Of interest to botanists is the fact that Parkinsonia is a drought-deciduous tree and performs a great deal of photosynthesis in its stems and petioles. Photosynthesis is conducted in leaves when they are present, however it is most efficient at photosynthesis after losing its leaves.
As a result of dry, hot conditions, Parkinsonia sheds all leaves (and in some instances petioles and young stems) during extended periods of stress and yet remains healthy and productive. Fewer resources are devoted to leaf maintenance during stress, allowing for health and stability while needed metabolites are produced in stems.
Drought-deciduous behavior may be changed by routine watering. It has been reported that when irrigated, Parkinsonia initially produces larger leaves than non-watered specimens. These large leaves remain highly functional, persisting for longer periods while water is available (Cannon 1905).
There is considerable variation among different species of plants that are drought-deciduous. Some plants, which are somewhat like Parkinsonia drop their leaves yet continue to perform photosynthesis in well-watered conditions. All photosynthetic activity is stopped, however, during drought. For example the shallow-root summer deciduous shrub Anthyllis (throughout Spain) performs most of its photosynthesis and vegetative growth during cool wet seasons (even if leaves have been dropped). With the onset of the dry, warm season, however, leaves are shed and photosynthetic rates decline to zero by mid summer (Haase et al. 2000). Interestingly, some drought-deciduous plants can perform stem photosynthesis while heavily lignified (Kocurek and Pilarski 2012).
Shallow-rooted plants such as Grayia (the saltbush) and Tetradymia (the short spine horsebush) drop leaves during summer drought, but, because of anatomical differences in xylem, they are able to retain and transport water during dry periods allowing for photosynthesis in stems (Hacke, Sperry, and Pitterman 2000). Often drought declining photosynthesis is due to cavitation (air embolisms) within columns of water retained in xylem tubes, interrupting water delivery. Many shallow-root, drought-deciduous plants suffer less from drought due to heavily lignified xylem tissues (Hacke, Sperry, and Pitterman 2000), which resist cavitation. Palo verde, a deep-rooted, drought-deciduous plant also features heavily lignified xylem (Scott 1935), adding another fitness feature to its list.
Lycium, (the boxthorn), a drought-deciduous shrub common in the Mojave Desert, produces short shoots from which microphyllous leaves are expressed early in the spring. These leaves have higher initial productivity than do leaves on long shoots. They are only produced during heavy rain years and supply much of the metabolites for annual growth (Hamerlynck et al. 2002). Thus in boxthorn, dropping leaves during periods of drought is complemented by the production of short shoots to continue photosynthesis. Productivity becomes much lower, however, after leaves are shed.
Still other drought-deciduous plants take advantage of intermittent rainfall during dry periods by retaining or producing non-dormant buds throughout the year. This allows the plant to rapidly flush or produce leaves following rainfall events (Jolly and Running 2004), only to drop them after dry periods return.
Drought-deciduous behavior in palo verde adds to its fitness for desert survival.
Stem Photosynthesis
Concurrent with drought-deciduous stress/survival behavior, much photosynthesis can take place in trunks, stems, and petioles of trees before and after their leaves are dropped. Many plants perform stem photosynthesis in a wide variety of habitats including Parkinsonia (or Cercidium as reported by Adams, Strain, and Ting, 1967). The majority of such plants seem restricted to desert or Mediterranean climates. As reported here, photosynthetic cells extended from the outer cortex of palo verde, well into vascular tissues, thus the stem anatomy of palo verde is fitted largely for photosynthesis. Stem photosynthesis is considered a significant alternative leaf photosynthesis. In fact 45% of total tree chlorophyll has been found in twig and branch chlorenchyma in some trees (Berveiller, Kierzkowski, and Damesin 2007; Kharouk et al. 1995).
It is certainly intuitive to expect that herbaceous or succulent plants with green stems and trunks perform photosynthesis in those organs as they do (Kocurek and Pilarski 2012; Nilsen and Sharifi 1997). Herbaceous green stems are also almost as efficient as leaves in the absorption of light for metabolic work (up to 90%—Kocurek and Pilarski 2012). Recent work shows that herbaceous plant stems do more than simply assist in light-related metabolic activities. Some herbaceous plants and trees capture light via stems and trunks and distribute the light internally through the vascular system—even to the roots (Sun et al. 2003; Sun, Yoda, and Suzuki 2005). It has also been reported that flowers and fruits perform photosynthesis as well (Aschan et al. 2005; Berveiller, Kierzkowski, and Damesin 2007).
There is no question that stem photosynthesis provides benefits for palo verde in stressed biomes. It extends the growth season for this plant in the absence of leaves and it efficiently recaptures and fixes carbon. Moreover, it lessens plant stress by providing a continuous supply of carbohydrates while minimizing the use of water during times of drought (Nilsen and Sharifi 1997; Pfanz 1999; Pfanz et al. 2002).
It is surprising, however, that highly lignified, wood plants such as alder, ash, aspen, beech, birch, oak, eucalyptus, ginkgo, pine, and certain other trees with seemingly opaque, hard bark perform stem photosynthesis even when the tree is not stressed at all by drought, pests, or pollutants (Cernusak and Hutley 2011; Kharouk et al. 1995; Kocurek and Pilarski 2012; Pfanz 1999; Pfanz et al. 2002; Wittman and Pfanz 2014). This is remarkable because these stems are not specialized for photosynthesis. Yet, as mentioned, stems in these lignified plants contain upwards of 45% of total tree chlorophyll (Berveiller, Kierzkowski, and Damesin 2007; Kharouk et al. 1995). We conclude that stem photosynthesis confers a fitness characteristic to palo verde in arid environments.
Other Anatomical Features
Sunken guard cells present on palo verde stems are thought to provide an advantage here for two reasons. Most plants feature guard cells on the surfaces of leaves which assist in gas exchange and reduction of water loss. But stem stoma are rare. (Lenticels take the place of stomates on stems of most woody plants.) The fact that guard cells are present and sunken into the stems of palo verde can be considered a fitness feature, because even on most leaves guard cells are not sunken in this way.
Hair cells (trichomes), present in significant numbers on palo verde leaves (figs. 3 and 4), petioles, and stems, are thought to interrupt air-flow over plant surfaces, to allow water absorption via contact, and to reduce loss of water in arid and other biomes (Wagner, Wang, and Shepherd 2004; Werker 2000). Many plants feature hair cells, so hair cells on palo verde stems might not be considered unique to plants in arid regions. Nevertheless they must certainly assist in water retention for palo verde and are considered a fitted feature for palo verde here.
Photosynthetic Efficiency of Two Distinct Photosynthetic Cycles
An exhaustive discussion of C3 and C4 photosynthesis systems exceeds the purpose of this paper, but it will be briefly covered here because of its presence in palo verde plants. Most plants, particularly the 165,000 dicot species, and a great many of the monocot grasses (Ehleringer, Cerling, and Helliker 1997), are known as C3 plants because they use atmospheric CO2 to manufacture a 3-carbon compound in leaves during photosynthesis. C4 terrestrial plants instead produce a 4-carbon compound within stems via an alternate pathway. It is considered a relatively new photosynthetic pathway evolutionarily speaking. It is generally accepted that C3 photosynthesis became the dominant terrestrial photosynthetic system before the C4 system developed, yet C4 metabolism has been found in unicellular oceanic algae and other less advanced [and ”earlier” plants], (Hibberd and Quick 2002; Reinfelder, Kraepiel, and Morel 2000). In any event, palo verde is a C4 photosynthetic plant.
C3 photosynthesis has been described as inefficient (Hibberd and Quick 2002; Moore 1982; Waller and Lewis 1979) because excess atmospheric CO2, unused during daytime photosynthesis, is later lost during photorespiration. Instead, C4 plants more efficiently capture and use chemically bound CO2 extracted from xylem and phloem tissues and produce more carbon compounds than do C3 plants. They also employ striking ultrastructural (anatomical) differences, which make remarkably efficient use of C4 metabolic pathways (Berveiller, Kierzkowski, and Damesin 2007; Gowik and Westhoff 2011; Muhaidat, Sage, and Dengler 2007; Waller and Lewis 1979). Some work has been done to support the idea that C4 photosynthesis developed in response to evergrowing arid environments (Gowik and Westhoff 2011; Hobbie and Werner 2004; Muhaidat, Sage, and Dengler 2007; Riebesell 2000). Gowik and Westhoff supply a creative stepwise chart of C4 evolution, but supporting data are absent within that, as well as the reports of others. Therefore arguments that C4 photosynthesis in most monocots and some dicots is due to convergent descent remain unconvincing. Moreover, the existence of many intermediates, which simultaneously employ C3 and C4 photosynthetic systems has been well established, (Gowik and Westhoff 2011; Hibberd and Quick 2002; Moore 1982; Muhaidat, Sage, and Dengler 2007). Reporting of empirical evidence demonstrating multiple convergences of the C4 photosynthetic pathway from the more established C3 pathway (32 independent convergences in dicots alone, i.e. Muhaidat, Sage, and Dengler 2007) would be useful. Regardless of such arguments, palo verde is convincingly fitted for success in arid conditions with C4 photosynthesis.
Moreover, plants subjected to drought have been shown to become severely limited in solar-induced chlorophyll fluorescence (SIF), using satellite imagery (Sun et al. 2015). (Chlorophyll autofluorescence, is a property of the molecule to give off infrared energy in the presence of sunlight.)
This diminished fluorescence is measurable on demand from space and is employed by workers to track pigment loss, thus monitoring drought severity across the country. A drop in SIF is considered to be an indicator of diminished chlorophyll production by plants under drought stress, particularly in summer months, when plants generally replenish their low chlorophyll supply. However, palo verde, like some other drought-resistant plants, maintains chlorophyll levels during drought periods, translating into high productivity in photosynthesis during drought (Arunyanark et al. 2008). This characteristic of palo verde we consider to be a compelling fitness feature during drought, which does cripple plants that cannot maintain levels of photosynthetic pigments under drought stress.
Stem Metabolic Deposits
Idioblasts are unusual plant cells, which have a secretory function and often store plant metabolites like oils, starches, minerals such as calcium oxalate, waste products, pigments, and other compounds in plant tissues. They reportedly have been found in all types of plant tissues (Coté 2009). They were also described in Parkinsonia leaves (Lersten and Curtis 1995, 2001), and we found them in abundance in Parkinsonia stems. Lerston and Curtis observed a lack of oils and crystals of calcium oxalate within idioblasts of palo verde leaves (Lersten and Curtis 1995), and they only saw small amounts of oil, and no starch or calcium oxalate in Parkinsonia stems, yet we found these also in abundance. Scott (1935) reported the presence of calcium oxalate crystals but only “at certain times.”
Calcium uptake by plants in excess of what is necessary and the deposition of calcium oxalate crystals has been widely reported (Franceschi 2001; Franceschi and Horner 1980; Franceschi and Nakata 2005; Lersten and Curtis 1995; Prychid, Jabaily, and Rudall 2008; Prychid and Rudall 1999; Webb 1999). It is largely unknown at present if calcium oxalate production and storage is solely a defense mechanism to inhibit herbivory or predation by other organisms (some needle like calcium oxalate crystals can even be ejected when disturbed, which might be painful when pushed into oral or other tissues [Coté 2009]). The formation of these crystals has been noted to play an important role in calcium regulation (Prychid, Jabaily, and Rudall 2008) and can also stiffen plant tissues (Coté 2009). It is proposed that they are useful in distribution of light within leaves because they are found in the palisade photosynthetic cells of leaves just under the epidermis (Franceschi 2001). Their use as a systematic guide is complicated by the fact that their presence is distributed among all taxonomic levels of plants from small algae to giant gymnosperms (Franceschi and Nakata 2005).
A significant note is that crystal growth is not controlled by random precipitation of oxalic acid with calcium ions; rather their size, formation, and distribution within plant tissues appears to be species specific and mediated by cellular control (Franceschi and Nakata 2005; Webb 1999).
The plentiful deposits of oil and starch in idioblasts of palo verde allows them to survive dry periods, another fitness character for the desert biome. The fact that druse and/or rosette crystals of calcium oxalate are the only forms observed in stems of this plant warrants further study.
Conclusions
The fitness characters of this interesting plant which thrives in hot, dry biomes are many. They include (but may not be restricted to) deep root penetration, microphyllous leaves, hair cell adornment, decumbent trunks, drought-deciduous behavior, petiole, stem, and trunk photosynthesis, thick cuticular covering on petioles, stems and trunks, sunken stoma, C4 photosynthesis, deep penetration of solar radiation into lignified stems, and trunks, (and vascular systems, which transmit the light deep into trunk tissues), lignified xylem to prevent cavitation, and production and storage of oils and starch as well as druse crystals of calcium oxalate. All these fitness characters in our estimation, serve palo verde well as it thrives in some of the most inhospitable areas on earth. We conclude that these characters are present due to planning and purpose of the Creator. However, well-vetted empirical data to the contrary are welcomed.
It also must be said that we heartily endorse the publication of novel field and laboratory-based research here. We believe that the reporting of design found within the tiniest structures of the creation is sufficient to point hearts to God and to lead to repentance (Romans 1:18–20). Our answer to the question, “Of what significance to the creation message is the science you are doing?” can be found in Paul’s exemplary pattern while he was at the Areopagus (Acts 17:16–ff). The creation message delivered by Paul from the steps of the highest court in Greece ended with the obvious: God’s will that “all people everywhere must repent.” Teaching creation should lead to repentance and submission to Christ even in our technologically minded society. Teaching creation must include novel research, produced by believers, and must speak to God’s invisible qualities. Lab and field research, from our own efforts (and not the work of others of the opposing worldview), carries authority and commands respect (especially among our detractors). These qualities are sadly missing (or severely minimized) in our work today.
People listening to Paul who were ignorant of God and His creation, were unaware (or at the least willfully ignorant) that articles made from “gold, silver, or stone” were not real gods. Tech oriented people today are smarter than that. But they respect novel science constructed using costly materials like world-class laboratories. The future of the creation movement lies not in museums or attractions or the library, but in the laboratory. The future of the creation movement lies in preaching the Gospel to the lost, those fields “white unto harvest” (John 4:35) who must be persuaded to repent and trust Jesus as Savior.
Acknowledgments
The authors are indebted to donors of the microscopy lab, including Per Larssen, Brent Fjarli, Matt Pierce, and George Howe. We also thank the anonymous reviewers and Andrew Snelling, all who made this a better manuscript.
References
Adams, Michael S., Boyd R. Strain, and Irwin P. Ting. 1967. “Photosynthesis in Chlorophyllous Stem Tissue and Leaves of Cercidium floridum: Accumulation and Distribution of 14C from 14CO2. Plant Physiology 42, no. 12 (December): 1797–1799.
Arunyanark, A., S. Jogloy, C. Akkasaeng, N. Vorasoot, T. Kesmala, R. C. Nageswara Rao, G. C. Wright, and A. Patanothai. 2008. “Chlorophyll Stability is an Indicator of Drought Tolerance in Peanut.” Journal of Agronomy and Crop Science 194, no. 2 (April): 113–125.
Aschan G., H. Pfanz, D. Vodnik, and F. Batic. 2005. “Photosynthetic Performance of Vegetative and Reproductive Structures of Green Hellebore (Helleborus viridis L. agg.). Photosynthetica 43, no. 1 (March): 55–64.
Berveiller, D., D. Kierzkowski, and C. Damesin. 2007. Interspecific variability of stem photosynthesis among tree species. Tree Physiology 27: 53–61.
Cannon, W. A. 1905. “On the Water-Conducting Systems of Some Desert Plants.” Botanical Gazette 39, no. 6 (June): 397–408.
Cernusak, Lucas A., and Lindsay B. Hutley. 2011. “Stable Isotopes Reveal the Contribution of Corticular Photosynthesis to Growth in Branches of Eucalyptus miniata.” Plant Physiology 155, no. 1 (January): 515–523.
Coté, Gary G. 2009. “Diversity and Distribution of Idioblasts Producing Calcium Oxalate Crystals in Dieffenbachia seguine (Araceae).” American Journal of Botany 96, no. 7 (July): 1245–1254.
Dimmitt, Mark A. 1987. “The Hybrid Palo Verde ‘Desert Museum’: A New, Superior Tree for Desert Landscapes.” Desert Plants 8, no. 3: 99–103.
Dimmit, Mark A. 2014. “Plant Ecology of the Sonoran Desert Region.” Arizona-Sonora Desert Museum. https://www. desertmuseum.org/books/nhsd_plant_ecology.php.
Ehleringer, James R., Thure E. Cerling, and Brent R. Helliker. 1997. “C4 Photosynthesis, Atmospheric CO2, and Climate.” Oecologia 112, no. 3 (October): 285–299.
Franceschi, Vincent. 2001. “Calcium Oxalate in Plants.” Trends in Plant Science 6, no. 7 (July): 331.
Franceschi, Vincent R., and Harry T. Horner. 1980. “Calcium Oxalate Crystals in Plants.” Botanical Review 46, no. 4 (October): 361–427.
Franceschi, Vincent R., and Paul A. Nakata. 2005. “Calcium Oxalate in Plants: Formation and Function.” Annual Review of Plant Biology 56: 41–71.
Gowik, Udo, and Peter Westhoff. 2011. “The Path from C3 to C4 Photosynthesis.” Plant Physiology 155: 56–63.
Haase, Peter, Francisco I. Pugnaire, S. I. Clark, and L. D. Incoll. 2000. “Photosynthetic Rate and Canopy Development in the Drought-Deciduous Shrub Anthyllis cytisoides L.” Journal of Arid Environments 46, no. 1: 79–91.
Hacke, Uwe G., John S. Sperry, and Jamila Pittermann. 2000. “Drought Experience and Cavitation Resistance in Six Shrubs from the Great Basin, Utah.” Basic and Applied Ecology 1, no. 1: 31–41.
Hamerlynck, Erik P., Travis E. Huxman, Therese N. Charlet, and Stanley D. Smith. 2002. “Effects of Elevated CO2 (FACE) on the Functional Ecology of the Drought- Deciduous Mojave Desert Shrub, Lycium andersonii.” Environmental and Experimental Botany 48, no. 2: 93–106.
Hobbie, Erik A. and Roland A. Werner. 2004. “Intramolecular, Compound-Specific, and Bulk Carbon Isotope Patterns in C3 and C4 Plants: A Review and Synthesis.” New Phytologist 161, no. 2: 371–385
Hibberd, Julian M. and W. Paul Quick. 2002. “Characteristics of C4 Photosynthesis in Stems and Petioles of C3 Flowering Plants.” Nature 415: 451–454.
Johnson, M. B. 1996. “Palo verde—A Review of the Genus Cercidium.” Aridus 8, no. 3 (August): 1–6.
Jolly, William M., and Steven W. Running. 2004. “Effects of Precipitation and Soil Water Potential on Drought Deciduous Phenology in the Kalahari.” Global Change Biology 10, no. 3 (March): 303–308.
Kharouk, V. I., E. M. Middleton, S. L. Spencer, B. N. Rock, and D. L. Williams. 1995. “Aspen Bark Photosynthesis and Its Significance to Remote Sensing and Carbon Budget Estimates in the Boreal Ecosystem.” Water, Air and Soil Pollution 82, no. 1–2 (May): 483–497.
Kocurek, Maciej, and Jan Pilarski. 2012. “Implication of Stem Structures for Photosynthetic Functions in Select Herbaceous Plants.” Polish Journal of Environmental Studies 21, no. 6: 1687–1696.
Lersten, Nels R., and John D. Curtis. 1995. “Two Foliar Idioblasts of Taxonomic Significance in Cercidium and Parkinsonia (Leguminosae: Caesalpinioideae). American Journal of Botany 82, no. 5 (May): 565–570.
Lersten, N. R., and J. D. Curtis. 2001. “Idioblasts and Other Unusual Internal Foliar Secretory Structures in Scrophulariaceae.” Plant Systematics and Evolution 227 no. 1–2 (May): 63–73.
Liogier, Henri Alain. 1990. Plantas Medicinales de Puerto Rico Y Del Caribe. San Juan, Puerto Rico: Iberoamericana de Ediciones Inc.
Moore, Peter D. 1982. “Evolution of Photosynthetic Pathways in Flowering Plants.” Nature 295 (25 February): 647–648.
Muhaidat, R., Rowan F. Sage, and Nancy G. Dengler. 2007. “Diversity of Kranz Anatomy and Biochemistry in C4 Eudicots.” American Journal of Botany 94, no. 3 (March): 362–381.
Nilsen, Erik T., and M. Rasoul Sharifi. 1997. “Carbon Isotopic Composition of Legumes with Photosynthetic Stems From Mediterranean and Desert Habitats.” American Journal of Botany 84, no. 12 (December): 1707–1713.
Pfanz, Hardy. 1999. “Photosynthetic Performance of Twigs and Stems of Trees With and Without Stress.” Phyton: Plant Physiology 39, no. 3: 29–33.
Pfanz, H., G. Aschan, R. Langenfeld-Heyser, C. Wittmann, and M. Loose. 2002. “Ecology and Ecophysiology of Tree Stems: Corticular and Wood Photosynthesis.” Naturwissenschaften 89, no. 4 (April): 147–162.
Polhill R. M., and J. E. Vidal. 1981. Caesalpinieae. In Advances in Legume Systematics, edited by R. M. Polhill and P. H. Raven, Part 1, pp. 81–95. Kew, United Kingdom: Royal Botanic Gardens.
Prychid, Christina J., and Paula J. Rudall. 1999. “Calcium Oxalate Crystals in Monocotyledons: A Review of their Structure and Systematics.” Annals of Botany 84, no. 6 (December): 725–739.
Prychid, Christina J., Rachel Schmidt Jabaily, and Paula J. Rudall. 2008. “Cellular Ultrastructure and Crystal Development in Amorphophallus (Araceae).” Annals of Botany 101, no. 7 (May): 983–995.
Reinfelder, John R., Anne M. L. Kraepiel, and François M. M. Morel. 2000. “Unicellular C4 Photosynthesis in a Marine Diatom.” Nature 407, no. 6807 (26 October): 996–999.
Riebesell, Ulf. 2000. “Carbon Fix for a Diatom.” Nature 407, no. 6807 (October 26): 959–960.
Rose, J. N., and Paul C. Standley. 1912. “Report on a Collection of Plants from the Pinacate Region of Sonora.” Contributions from the United States National Herbarium 16, no. 1: 5–20.
Scott, Flora Murray. 1935. “The Anatomy of Cercidium torreyanum and Parkinsonia microphylla.” Madroño 3, no. 2 (April): 33–41.
Scott, Flora Murray, B. G. Bystrom, and E. Bowler. 1962. “Cercidium floridum Seed Coat, Light and Electron Microscopic Study.” American Journal of Botany 49, no. 8 (September): 821–833.
Schuch, Ursula K., and Jack J. Kelly. 2008. “Palo Verde Trees for the Urban Landscape.” Aridus 20, no. 1: 1–6.
Schuch, Ursula K., and Jack J. Kelly. 2012. “Mesquite and Palo Verde Trees for the Urban Landscape.” University of Arizona College of Agriculture and Life Sciences, Arizona Cooperative Extension Bulletin AZ1429.
Sun, Qiang, Kiyotsugu Yoda, and Hitoshi Suzuki. 2005. “Internal Axial Light Conduction in the Stems and Roots of Herbaceous Plants.” Journal of Experimental Botany 56, no. 409 (1 January): 191–203.
Sun, Qiang, Kiyotsugu Yoda, Mitsuo Suzuki, and Hitoshi Suzuki. 2003. “Vascular Tissue in the Stem and Roots of Woody Plants Can Conduct Light.” Journal of Experimental Botany 54, no. 387: 1627–1635.
Sun, Ying, Rong Fu, Robert Dickinson, Joanna Joiner, Christian Frankenberg, Lianhong Gu, Youlong Xia, and Nelun Fernando. 2015. “Drought Onset Mechanisms Revealed by Satellite Solar-Induced Chlorophyll Fluorescence: Insights From Two Contrasting Extreme Events.” Journal of Geophysical Research: Biogeoscience 120, no. 11: 2427–2440.
Wagner, G. J., E. Wang, and R. W. Shepherd. 2004. “New Approaches for Studying and Exploiting an Old Protuberance, the Plant Trichome.” Annals of Botany 93, no. 1 (1 January): 3–11.
Waller, S. S., and J. K Lewis. 1979. “Occurrence of C3 and C4 Photosynthetic Pathways in North American Grasses.” Journal of Range Management 31, no. 1: 12–28.
Webb, Mary Alice. 1999. “Cell-Mediated Crystallization of Calcium Oxalate in Plants.” The Plant Cell 11 (April): 751– 761.
Werker, E. 2000. “Trichome Diversity and Development.” Advances in Botanical Research 31: 1–35.
Wittmann, Christiane, and Hardy Pfanz. 2014. “Bark and Woody Tissue Photosynthesis: A Means to Avoid Hypoxia or Anoxia in Developing Stem Tissues.” Functional Plant Biology 41, no. 9: 940–953.